Changes in the shape of the shoot apex are synchronized with floral transition in Arabidopsis
As plants initiate flowering, the shoot tip enlarges and undergoes genetic reprogramming. However, how these changes in shoot-tip shape are co-regulated with the floral transition is unknown. In a new study published in Nature Communications, researchers from the group of George Coupland at the Max-Planck Institute for Plant Breeding Research in Cologne, Germany, show that the reciprocal repression of two genes at the plant apex synchronises changes in meristem shape with the floral transition in the model plant Arabidopsis thaliana.
Thale cress (Arabidopsis thaliana) plants transition from vegetative growth to flowering when exposed to long days of spring and summer. In response to this environmental cue, the tissue at the plant apex, known as the shoot apical meristem (SAM), undergoes genetic reprogramming to produce flowers instead of leaves. At the same time, the SAM increases in size and elongates to form a characteristic domed shape. It is unknown how changes in SAM shape occur during floral transition; therefore, the authors first studied in detail how the internal organisation of the SAM changes during the floral transition. The SAM contains two major sub-domains, and its size is regulated by interactions between proteins that are characteristically expressed in these regions. The authors used two fluorescent reporter markers specific to these two domains and imaged their expression over time as plants began to flower. A computational pipeline that quantified the intensity of these fluorescent proteins in groups of cells relative to the shoot apex showed precisely how the increases in overall SAM width and height during floral transition correlated with changes in the size of the internal domains. They found that particularly the central zone at the very tip of the SAM increased in height and width, and that the peripheral zone on the flanks of the SAM increased markedly in width during the floral transition.
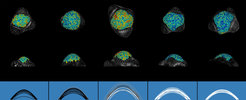
The authors then focussed on the AP2 gene, which encodes a transcriptional regulator that promotes doming of the SAM but also represses flowering. The amount of AP2 protein in the SAM is high before flowering and becomes progressively depleted. If the time during which AP2 is present is extended, the width of the SAM increases. To identify how AP2 regulates the changes in SAM morphology during floral transition, the authors compared the genes that were expressed in SAMs that either express AP2 or that lack AP2, and compared the resulting differentially expressed genes with those that are known to be directly regulated by AP2. By this approach, they identified the SOC1 gene, which encodes another class of transcriptional regulator, and characterised its pattern of protein expression in the SAM compared with that of AP2 during the transition to flowering. The proteins show opposite patterns of accumulation throughout floral transition, with AP2 gradually disappearing whilst SOC1 gradually accumulates, which is consistent with flowering being repressed by AP2 and promoted by SOC1. Moreover, mutants lacking SOC1 have a large SAM, and SOC1 accumulates more in the SAMs of plants lacking AP2, indicating that AP2 represses SOC1 production. These observations, combined with the ability of both proteins to bind directly to each other’s genes, suggests that mutual repression of AP2 and SOC1 in the SAM plays a role in integrating changes in SAM size with flower production.
A mutual feedback model explains how SAM morphology and flowering are correlated
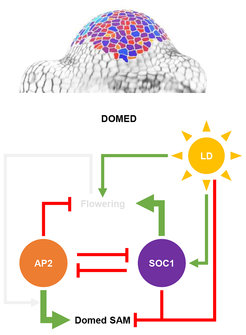
The authors combined their observations into a model that neatly accounts for how AP2 and SOC1 synchronise changes in SAM morphology with flowering time and flower production. During vegetative development, AP2 delays floral transition, in part by repressing the SOC1 gene, but does not influence SAM morphology. When plants are induced to flower by long days, SOC1 increases in abundance and in turn, represses the production of AP2 and promotes flowering. Before AP2 disappears completely, it promotes increases in SAM height and width, and its disappearance ensures that the SAM does not excessively increase in width. A central feature of the model is the importance of mutual repression of AP2 and SOC1, which determines the time interval during which both factors are expressed. This determines the timing of flower production and limits the stage during which AP2 promotes changes in SAM morphology. This type of direct reciprocal repression has been characterized in developmental processes in animals, and the study helps to underscore how developmental decisions in plants are triggered by environmental signals that induce gene regulatory networks expressed in robust spatiotemporal patterns.